ANITA™Mox – Lessons Learned from a Year of Deammonification
Katya Bilyk, Wendell Khunjar, Greg Pace, Thomas Worley-Morse, Simon Lobdell, Ron Taylor, Bob Gasper, and Paul Pitt
Last Modified Sep 19, 2022
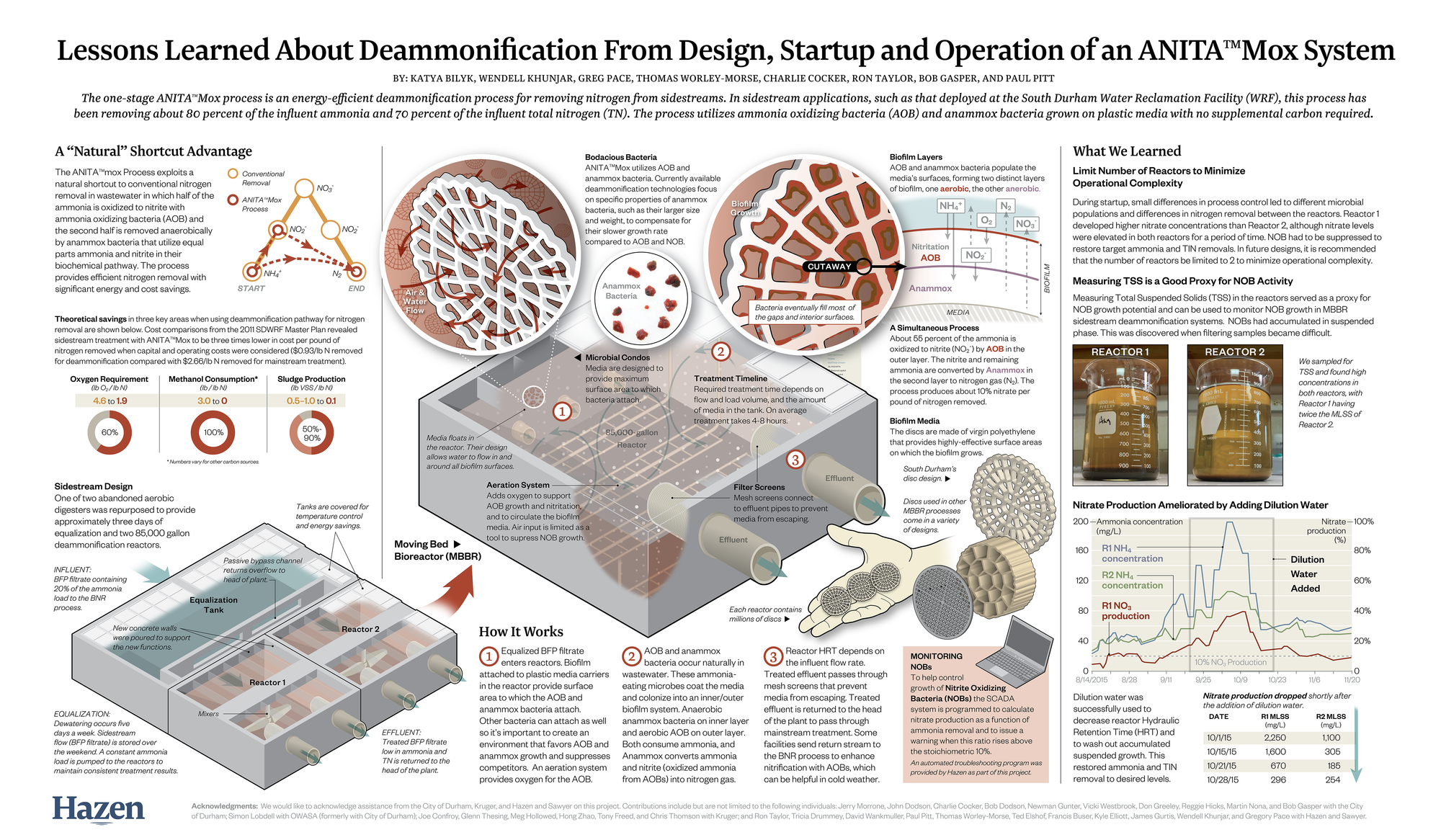
Introduction
In 2011, the City of Durham completed a comprehensive wastewater master planning effort. The SDWRF will have to meet a total nitrogen limit of 3 mg/L and a total phosphorus limit of 0.23 mg/L at its 20-mgd design flow to comply with the total maximum daily load limits in its Jordan Lake Watershed. As part of the study, sidestream treatment for nitrogen removal was recommended. Sidestream nitrogen load at the SDWRF is about 20 percent of the load to the biological nutrient removal process. Cost comparisons with mainstream treatment revealed sidestream treatment using the deammonification pathway to be three times lower in cost per pound of nitrogen removed when capital and operating costs were considered ($0.93/lb N removed for deammonification compared with $2.66/lb N removed for mainstream treatment).
At the SDWRF, one of two abandoned aerobic digesters was repurposed to provide approximately three days of equalization and two 85,000 gallon deammonification reactors. The ANITA™Mox process by Kruger was selected. Design was completed in 2013, and the construction began in 2014. The ANITA™Mox process started up in May 2015 and was fully treating all of the filtrate load at the SDWRF by November 2015.
Results
Both reactors were started in parallel. Seed media was brought in from the James River Treatment Plant in Virginia, and the reactors were seeded at seven percent of the installed media by volume. Initially, the seeded media was fed filtrate in batches. The reactors were then transitioned to continuous flow when the seeded media could treat the nitrogen load provided by the pumps at their lowest continuous flow rates.
Continuous aeration mode has not yet been initiated because the minimum mixing airflow is slightly higher than the process air demand, so intermittent aeration is used. The current aeration cycle is approximately 50 minutes of aeration followed by 10 minutes of mixing. Operating in continuous aeration mode may be possible in the near future, and will allow treatment in a single phase aerobic mode with a low dissolved oxygen setpoint.
Nitrate, nitrite, orthophosphate, ammonia, chemical oxygen demand (COD), and alkalinity were measured several times per week with grab samples to monitor the progress of the process. Weekly conference calls between the City of Durham, Kruger, and Hazen were conducted during startup.
Significant Findings
- Small variances in process controls can lead to different microbial communities and differences in nitrogen removal—therefore for future designs no more than two reactors would be recommended to minimize operational complexity;
- Measuring TSS in the reactors served as a proxy for NOB growth potential and can be used to monitor NOB growth in MBBR sidestream deammonification systems; and
- Dilution water can be used to decrease HRT and wash out suspended NOB growth.
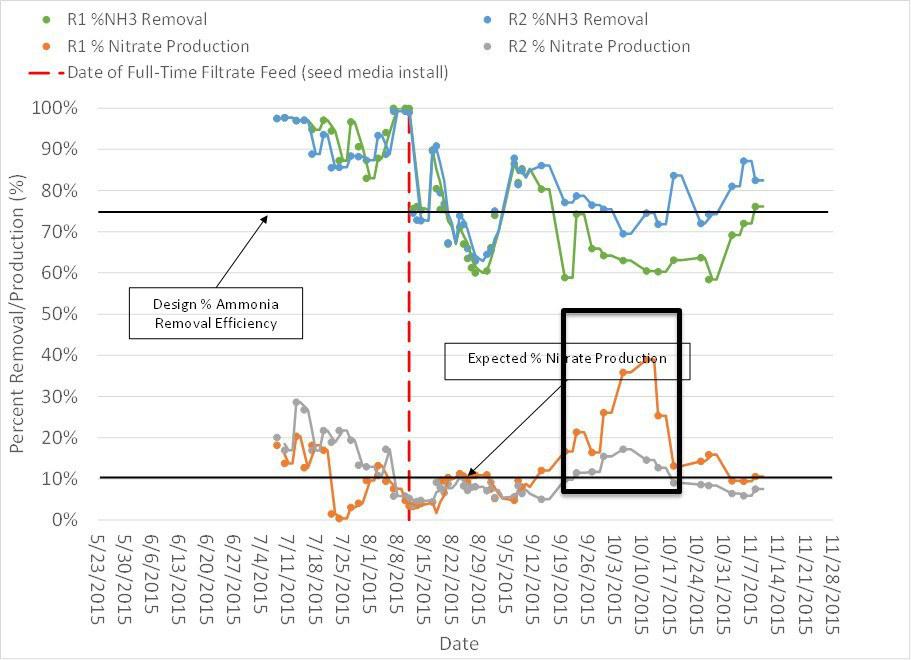
Figure 1 shows the percent ammonia removal in each reactor and the nitrate produced as a ratio of the amount of ammonia removed. The process is currently removing greater than 80 percent of the influent ammonia to the reactors. Ideally, the nitrate production ratio should be less than ten percent per deammonification reaction stoichiometry. Higher ratios of nitrate to ammonia suggest the presence of nitrite oxidizing bacteria (NOB), which compete with the anammox bacteria for nitrate and reduce the efficiency of the nitrogen removal process.
In Figure 1, the box highlights when the nitrate to ammonia production rate increased above the desired rate of 10% for a period of time and that the problem was worse in Reactor 1. This experience points out that different populations can develop in the reactors and this is an important consideration for design and operational complexity.
The cause of the rise in nitrate production during startup was investigated. The reactors started to diverge when the reactors were operated in a two-phase batch feeding regime, an aerobic phase and an anoxic mixing-only phase. During this time, the sampling team began observing that the solids from Reactor 1 (R1) were more difficult to filter for dissolved nutrient analysis than the solids from Reactor 2 (R2). This prompted a comparison of the total suspended solids (TSS) concentrations between the two reactors, and it was revealed that R1 had nearly twice the solids concentration that R2 did. Although there were no major operational differences between the two reactors at this time in the startup, there were a few minor differences in the mixing, flows, and aeration that likely contributed to the increased suspended solids in R1. These slight differences and their design implications will be discussed in greater detail in the final manuscript.
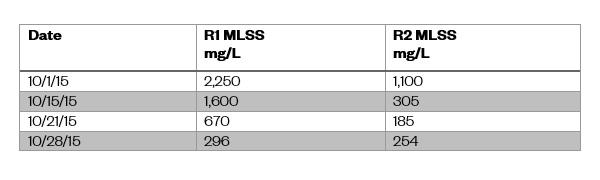
During the weekly conference call the occurred in this period, Kruger suggested that the hydraulic retention time (HRT) in the reactors was too long and that dilution water (UV-disinfected plant effluent) be added to flush out the NOBs that had accumulated in both reactors with R1 requiring more dilution water than R2. Table 1 shows how this approach was successful in reducing the suspended phase concentration of solids. Shortly after October 28, 2015, the final date where TSS sampling is available, the nitrate to ammonia production rate dipped below 10% in both reactors (Figure 1). Therefore, this strategy was successful. The ratio of dilution water to total flow was approximately 1:4. Although temperature was not an issue at this time of year, it should be noted that supplemental heating could potentially be needed if dilution water to flush out NOBs is incorporated into a full-scale design. The SDWRF has the ability to heat the equalization basin contents using excess heat from the anaerobic digesters that runs through heating coils along the floor.